Volume 2
Chemistry, theoretical, practical, and analytical : as applied and relating to the arts and manufactures / by Dr. Sheridan Muspratt.
- Muspratt, Sheridan, 1821-1871.
- Date:
- [1860]
Licence: Public Domain Mark
Credit: Chemistry, theoretical, practical, and analytical : as applied and relating to the arts and manufactures / by Dr. Sheridan Muspratt. Source: Wellcome Collection.
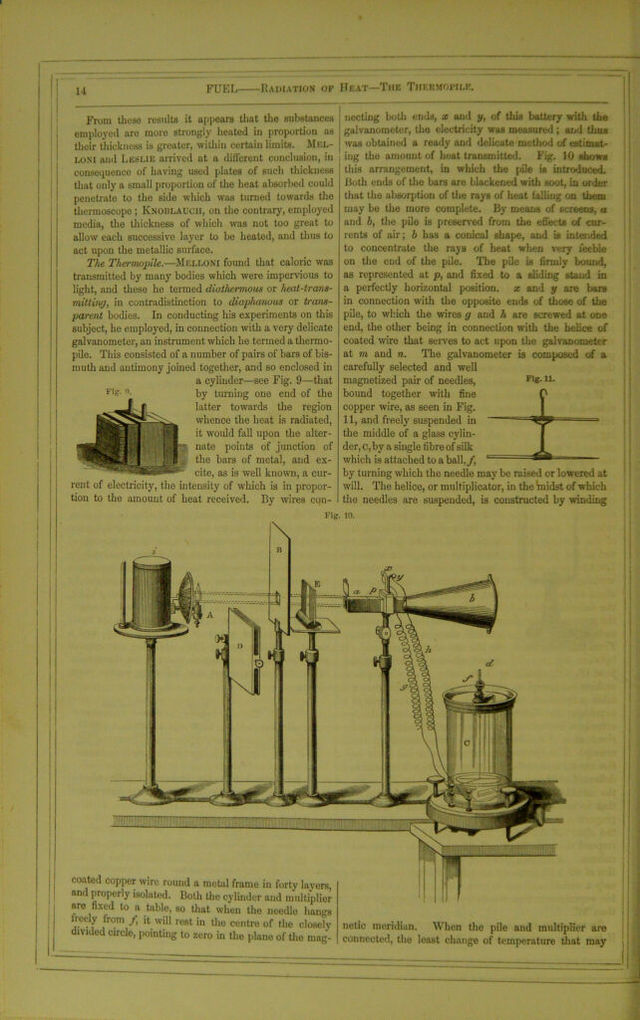
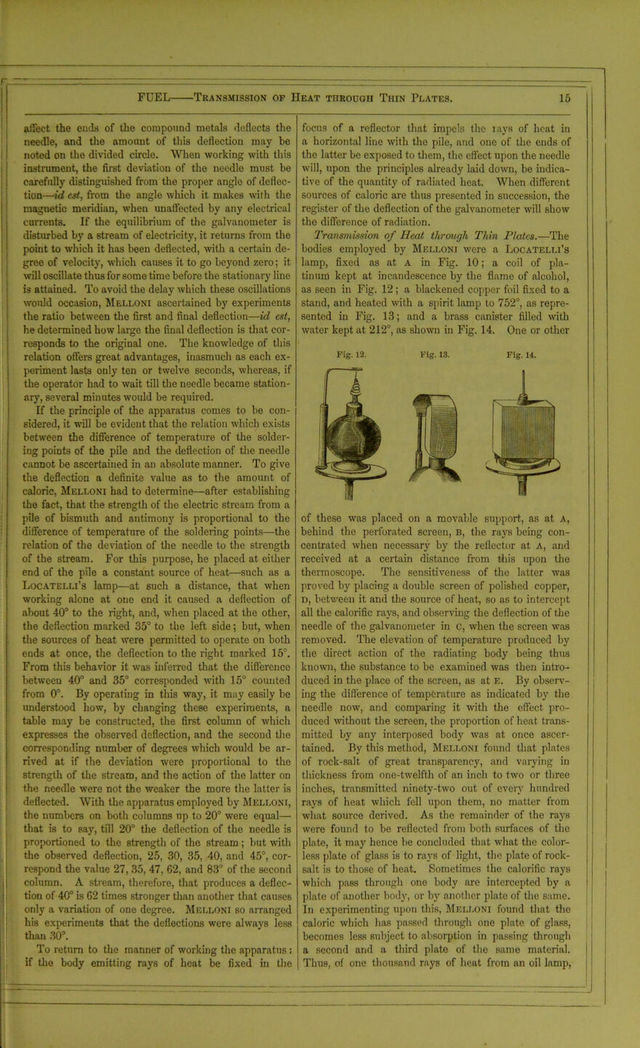
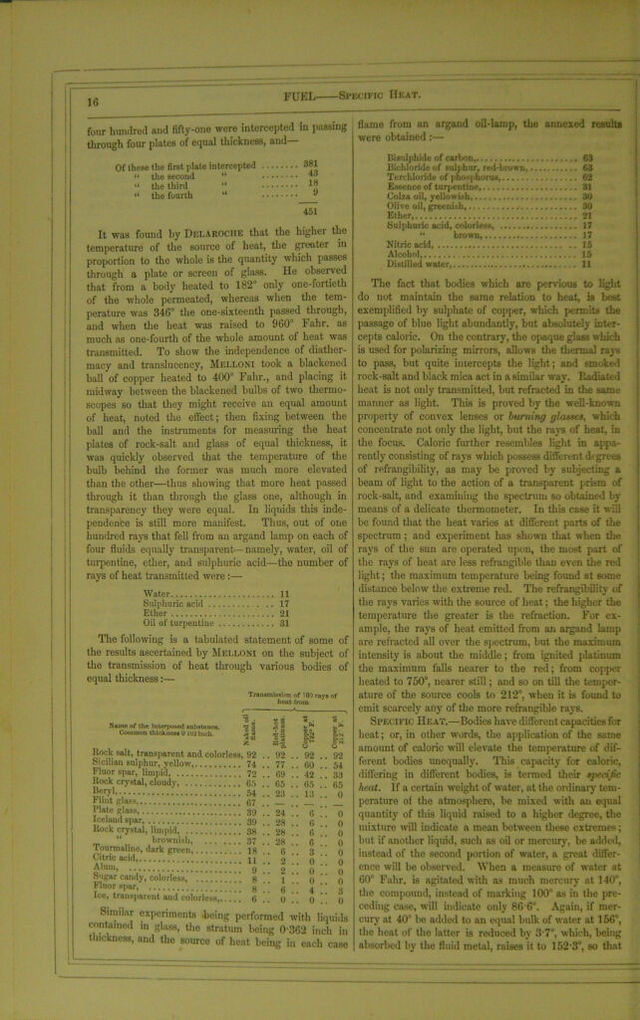
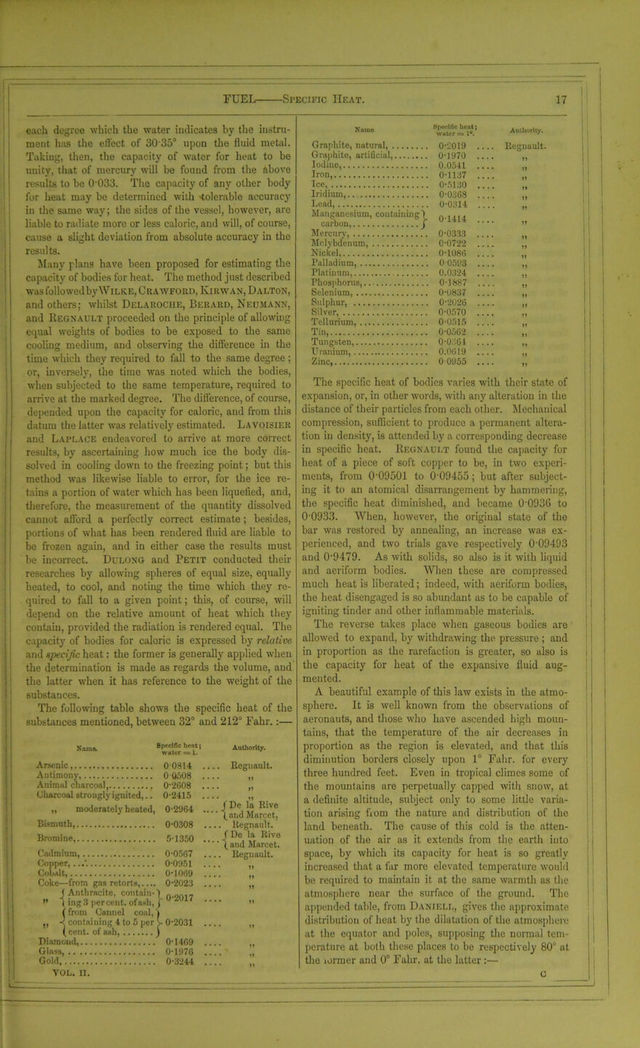
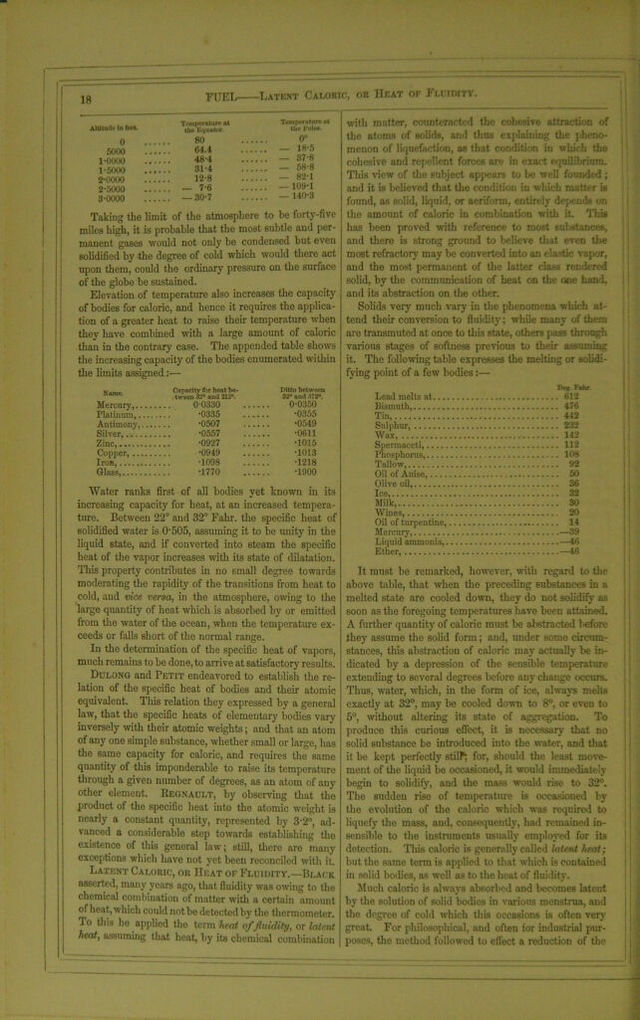